The Evolution of Lipid Nanoparticles: From Biophysics to Gene Therapy, Vaccines and Drug Delivery
On April 27th Keystone Symposia hosted a free ePanel to explore recent breakthroughs in nanoparticle technology, design and applications. Featuring field pioneers Dr. Philip Felgner, Dr. Terry Allen and Dr. Pieter Cullis, we explored the evolution of the field from inception to modern day, covering advances that have enabled the COVID-19 mRNA vaccines, gene therapy and novel cancer treatment options.
Read the event summary below to discover how the field evolved from basic science to clinical medicine, what were the biggest challenges along the way, and where the field is headed now in the age gene therapy and mRNA vaccines. Finally, the panelists share their advice for the next generation of scientists who will follow in their footsteps and take the field to new heights.
Watch the free ePanel Event Here
In collaboration with:
The Evolution Of Lipid Nanoparticles: From Biophysics To Gene Therapy, Vaccines And Drug Delivery
By Shannon Weiman
Dr. Pieter Cullis was drawn to the field of lipid nanoparticles 50 years ago, when very little was known about these ‘magic bullets.’ Coming from a background in physics, he was intrigued by the complexity of biological systems, and applied his knowledge to study the physical properties of lipids in biological membranes. He examined lipid asymmetry, and also why some lipids would form bilayer membranes, while others would adopt different structures like micelles.
In parallel, Dr. Philip Felgner was also studying the biophysics of small unilamellar vesicles (SUVs), and how lipids assembled into these physically stable, self-assembled systems as a first step to being able to engineer them for pharmaceutical applications. He discovered that the fatty-acid chains of the lipid molecules dictated the structural and physical properties like shape, viscosity and stability. Longer and more saturated fatty-acid tails made for more waxy and stable SUVs, whereas unsaturated and shorter fatty-acids were more oily, and disintegrated rapidly when they came into contact with biological membranes. This knowledge enabled scientists to engineer liposomes with the desired properties for clinical applications.
Once scientists could engineer the lipids to their specifications, the next big step was how to deliver cargo such as DNA, mRNA or drug molecules. Both Dr. Felgner and Dr. Cullis applied their knowledge of physics and electrostatic forces to devise strategies that deployed positively charged, cationic lipids to attract negatively charged nucleic acid molecules.
Dr. Felgner was working for Syntex, a company focused on vaccine development, and was looking to deliver very large pathogen-derived DNA molecules (5,000 base pairs at 1700 nm long), which could not fit within the 70nm diameter LNPs. Instead, he attempted to create an aggregate by mixing the DNA with cationic LNPs, which might be taken up in to cells as a clump. Although no aggregate was visible, he decided to add the mixture (now known as a “lipoplex”) to cultured cells anyway to see what would happen, and miraculously, it worked! The cells took up the DNA, which cannot cross the cell membrane on its own due to its size and negative charge, and started producing the protein product. And thus “lipofection” was born. Dr. Felgner optimized the formulation and commercialized the Lipofectin Reagent in 1987—which has now become an essential tool in laboratory research and the mostly widely used method for cell transfection.
Examining the structure of the lipoplex in more detail, Dr. Felgner found that the nucleic acid acted as a scaffold, compacting in the center of the structure, and encapsulated by the lipids on the outside, making for its efficient uptake into cells. This synthetic virus-like particle was also remarkably stable due to the strength of the electromagnetic interactions, allowing it be frozen and thawed without losing efficacy—paving the way for vaccine applications.
Gene Therapy Advances
However, the success of these lipoplexes in vitro, did not translate in vivo; the positively charged lipids bound to the extracellular matrix, preventing uptake into cells. In addition, although the cationic liposomes themselves were well tolerated in animals, when mixed with DNA they became inflammatory, causing unwanted immune reactions. Faced with these challenges, Dr. Cullis devised ionizable cationic lipids to solve these in vivo problems. These lipids are positively charged at pH4, at which point the nucleic acids can be packaged into LNPs. Then upon transition to pH7, the lipids lose their positive charge, but retain their cargo, thereby creating non-charged, relatively non-toxic LNPs for the delivery of nucleic acids. This advance enabled gene therapy approaches to deliver mRNA or siRNA to produce or inhibit the production of a target protein. Having addressed the safety issue, Dr. Cullis co-founded a company co-founded focused on packaging siRNA and then mRNA in LNPs for gene delivery starting in 2005.
Since then, Dr. Cullis and his associated companies have synthesized approximately 700 different species of ionizable cationic lipids, each with different pharmacokinetic properties, and vastly different abilities to transfect cells by enhancing the ability of siRNA and mRNA to escape from endosomes.
With the ionizable lipids we really did hit a bit of a gold mine because it not only allowed us to encapsulate nucleic acids, but also to enhance intracellular delivery, and then not only that, but the ionizable lipid provides a very good adjuvant in the case of vaccines. So that has been one of the most interesting stories here, says Dr. Cullis.
In 2018 the first LNP based gene therapy was approved by the FDA, called Onpattro, for the treatment of hATTR Amyloidosis—a disease that resulted from mutant protein production in the liver. The LNPs delivered siRNAs that were capable of halting the production of the problematic protein. The treatment was spectacularly successful, offering stabilizing and possibly curative therapy for a previously fatal disease.
This is just the tip of the iceberg, says Dr. Cullis, who envisions LNP enabled gene therapy approaches against diseases from cancer to heart disease to neurodegenerative diseases, in addition to the traditional targets of genetically inherited diseases, like cystic fibrosis, sickle-cell anemia and Huntington’s disease.
Vaccine Advances
After the success of his “lipoplexes” in vitro, Dr. Felgner moved to in vivo testing, injecting his lipoplexes intramuscularly in animal models, the same route of administration that vaccine delivery would use. To his surprise, it also worked the first time, and the muscle cells expressed the encoded protein at high levels. This was the first proof of principle that LNPs could be a viable platform for vaccine antigen delivery.
Eager to take the next step towards clinical utility, he developed a collaboration with Merck to work toward LNP-based influenza vaccines. Deploying nucleocapsid DNA as the cargo, they were able to demonstrate heterologous protection against influenza, but were never fully successfully due to both the challenging viral targets they prioritized back then, as well as the lack of range of lipid nanoparticles available today. However, the clinical development and advances they accomplished during this period, paved the way for successful COVID-19 mRNA-based vaccines. In the meantime, he was able to develop an LNP-based vaccine adjuvant, that incorporated bacterial cell wall components into liposomes to induce strong immune responses, demonstrating their immunogenic potential.
At the time, Dr. Cullis co-founded a company called Acuitas Therapeutics, focusing on the concept of gene therapy by delivering mRNA to the liver to express therapeutic proteins. Acuitas was surprised when Dr. Katalin Kariko, of BioNtech, and Dr. Drew Weissman approached them in 2014 about adapting their LNPs for use as vaccines. They had developed a technique to utilize mRNA to produce vaccine antigens, but were lacking an efficient delivery system—Acuitas had just the solution.
We weren’t thinking about vaccines at all until they approached us- we were going after expression in the liver. The serendipity of science is really powerful, says Dr. Cullis.
Teaming up, they worked on influenza and Zika virus vaccines first, and in 2017 published on their highly effective mRNA vaccines that coded for Zika envelope proteins. They were working with BioNtech on the mRNA based influenza vaccine when COVID-19 emerged in 2020. They quickly transitioned and by November had completed the phase 3 study demonstrating 95% efficacy with the mRNA-LNP based vaccine—an unprecedented success in terms of protection and timeline, which was facilitated by 50 years of basic science and translation research on LNPs.
By this time, Dr. Felgner had moved to vaccine antigen discovery at UC Irvine, having developed a high throughput microarray system to efficiently identify the targets of protective antibodies found in human serum. This information could then be used to guide vaccine antigen development, as well as antibody therapeutics. When COVID-19 came along, he adapted the system for surveillance of immunity induced by vaccination, testing the serum of vaccinated health care workers for protective antibodies. When he discovered that 78% of patients were seropositive for protection only 1 month after vaccination, which then rose to 93% after boosting, he was shocked at the spectacular immune responses induced by the mRNA vaccines.
Part of this efficacy is likely due to the fact that once liposomes are injected into the body, they are naturally brought to lymph nodes—tissues that are built to optimize immune responses and immune memory, says Dr. Felgner. However, there is still room for improvement, he says, and further study of lipid structure-function relationships could help to reduce the strong side effects of the vaccine. “We might be able to engineer them differently to minimize the side effects while still maintaining the immunogenicity,” says Dr. Felgner. Dr. Cullis agrees, the field has relied on the remarkable adjuvant properties of LNPs to potently stimulate the immune system, without looking into how they might further manipulate or guide that immunogenicity. “If we actually focus on studying the immune response aspects of LNPs, we could make even better vaccines,” he says
Building upon the initial success of the COVID-19 mRNA vaccines, Dr. Felgner is enthusiastic about what this means for vaccine development in general. Adapting the LNP-based vaccine approach against other pathogens could yield much more effective protection against other viruses, bacteria, and parasites, including those for which there are currently no vaccines. In addition, there is potential do develop vaccines against non-microbial diseases for which there are no cures, such as cancer and neurodegenerative diseases.
Cancer Drug Delivery Advances
Meanwhile, Dr. Theresa M. (Terry) Allen was working on developing LNPs for delivering drugs like chemotherapy agents into cells. Back in the 1980s, LNPs faced 2 major challenges as drug carriers. First, they were rapidly removed from circulation as foreign bodies, which Allen solved by coating the surface with monosialoglycoprotein GM1 and later polyethylene glycol (PEG). These substances shield the LNPs from opsonization and phagocytosis, while preventing aggregation, substantially prolonging their circulation time.
Second, loading drug molecules into LNPs was inefficient, while leakage out of the LNP was high. Drs. Barenholz and Cullis solved this problem for anticancer drugs that were weak bases by creating pH gradients across the LNP bilayer, resulting in efficient uptake and improved retention of drugs into the LNPs.
Together, these advances led to the successful development of the first LNP-based anticancer drug, Doxil, approved by the FDA in 1995. With sales now in the 1,245 million range annually, Doxil is the poster child for drug delivery using liposomes.
In fact, Dr. Allen proved that delivering anticancer treatment in liposomes can reduce toxicity, compared to delivering the drug alone. As the field of cancer medicine has moved toward combination therapies to improve efficacy while reducing toxicity, LNP-based therapies have followed suit. The first LNP combination therapy was approved by the FDA in 2017, called Vyxeos which packaged daunorubicin and cytarbine together, to treat Acute Myeloid Leukemia (AML).
Dr. Allen was also able to improve LNP drug delivery, and thereby efficacy, by enhancing the internalization of liposomes into target cells through ligand-targeted delivery. By understanding the biological mechanisms behind phagocytosis, she was able to engineer the placement of ligands such as antibodies on the exterior of the LNP, which trigger the internalization of LNPs into the target cells. This significantly improved the selective intracellular delivery of drugs into cancer cells where they act intracellularly.
This strategy has since been adapted using different ligands and mechanisms for endocytosis that have emerged from basic biology studies. All three panelists agree that further investigation of endocytic mechanisms will enable the field to engineer more versatile and targeted approaches for ligand-targeted delivery of drugs, as well as gene therapies.
Indeed, all of Dr. Allen’s advances have improved the bioavailability and pharmacokinetic properties of LNPs for not only drug delivery, but across all modalities, from gene delivery to vaccines.
LNPs have opened the door to treat genetic, diseases, rare diseases, neglected diseases and currently incurable diseases, she says. The sky is the limit!
Future Directions
The next and ongoing challenge in the field is how to selectively target LNPs to specific cell types or organs, beyond the liver and lymph nodes where they naturally accumulate. Dr. Allen has already made strides towards this ‘holy grail,’ as she calls it, successfully targeting solid tumors outside the liver to deliver chemotherapy agents and gene therapies in 2011. However, translating this success from animal models to humans is no easy task.
Just this year those possibilities have expanded, and Dr. Drew Weissman’s group was able to target T cells specifically for CAR T cell therapy in mice (Dr. Drew Weissman- https://www.science.org/doi/10.1126/science.abm0594), expanding the repertoire of targeted approaches. Dr. Allen notes that specifically targeting immune cells that are circulating in the blood and lymphatic system is the most logical next step, as these cells are most easily accessible to LNPs, whereas targeting cells for example in the brain, will be much more challenging.
"If you can hijack natural processes it makes your job of targeting much easier,”says Dr. Cullis. Whether we will be able to do that for other tissues is an open question, but I do think the possibility is there.
Dr. Felgner agrees that taking advantage of natural targeting mechanisms is the key. We are already doing so for vaccines, including the LNP-COVID vaccines, which naturally target lymph nodes after intramuscular injection without requiring special engineering to do so. However, targeting other tissues and cell types will require new knowledge. Dr. Felgner points to new technologies that enable visualization of living cells as critical tools for discovering new mechanisms for bioengineer targeted LNPs. “Intravital imaging capabilities will be very informative, allowing us to see cellular movements and interactions in real time on a television screen. We haven’t even begun to look at these systems in that way yet and when we do it is really going to open our eyes,” he says.
Dr. Allen adds that not only should we be looking at engineering mechanisms to target uptake of LNPs into specific cell types, but also to optimize the release of cargo once the LNP has been endocytosed. Charged lipids are one way to trigger this intracellular release, but there may be others. Dr. Cullis agrees we need to understand the biology of intracellular release better in order to optimize efficacy. “This is an area of basic science that we really need to focus on,” he says.
As we envision these advances, it is also important to recognize that as the LNPs become more complex, it will become more challenging to mass produce them for clinical use. “We need to think in terms of use not only in the lab, but manufacturing and scaling up production of those systems,” says Dr. Allen.
Advice for the Next Generation
Wrapping up the panel we asked these field pioneers how they were drawn into the field when it was just forming, and what advice they have for the next generation of scientists, just starting out in this now exploding area of research.
Dr. Pieter Cullis
Coming from a physics background, Dr. Cullis was an anomaly in the biological world.
“The complexity of biology usually scares the hell out of physicists, but I found it intriguing and it motivated me to apply my knowledge to new challenges. It gives you a real benefit to change fields- you bring a lot to the new field with your unique background—my physics background in the end was quite useful, which may not have been obvious in the beginning.”
Advice for the Next Generation
“If you want to get into a rapidly growing area of therapeutics, then gene therapy is really exciting right now and the range of potential applications is just unbelievable. Almost any disease you can name you can think of using an siRNA or mRNA gene therapy approach. Virtually any disease you can invent a medicine for in a matter of months or less, once you know the protein you want to make or silence- it’s just incredible! So for younger people this is definitely an area of great excitement and growth. This is a wonderful area of science to get into right now.”
Dr. Philip Felgner
Dr. Felgner also stepped into the field from a biophysics background, recruited by a pharmaceutical company to adapt LNPs for drug delivery. He learned a lot by switching fields and was also able to contribute a lot by doing so, encouraging others to do the same.
"They weren’t interested in the biophysics, they were interested in solving problems, but the biophysics that we learned helped us solve those problems that others who didn’t have the biophysics training would not have been able to solve.”
Advice for the Next Generation
“Although it’s taken so many years to get here, what we experienced from this episode is the continuity of science—vaccine development has well over 100 years of background that we are building on. It has taken so many developments and so much maturation and thousands of people to build on what was learned in the past. Finally this field has matured to this level and we are only at the beginning of our opportunity to take advantage of it. It’s a great time to enter the field because everyone is at the starting line of this technology.”
Dr. Theresa Allen
Dr. Allen came from a marine biology background, as an expert scuba diver collecting samples for drug discovery. When working on a project examining the acetylcholine receptor in rays, she had to reconstitute the protein in a lipid bilayer to study its structure and function. Instead, she fell in love with the lipid bilayer, and the rest is history.
Advice for the Next Generation
“Be open minded, be imaginative—know what has happened in the past but don’t let it be a crutch preventing you from thinking creatively in new directions about what might happen in the future. Quite often what we know can constrain our thinking about what we can do outside the box. Try to think outside the box because you never know where it will take you. Fifty years ago we would not have predicted the current path to vaccines for emerging viruses; predictions tell us more about the past context than future potential. Your training shows you how to think and do research, and once you learn that you can pretty much do anything you set your mind to.”
Watch the free ePanel Event On Demand on the Keypoint Blog HERE
In collaboration with:
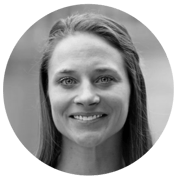
Related news
Continuing the Conversation on Lipid Nanoparticle Technologies for Novel Vaccines and Therapies
On April 27th Keystone Symposia hosted a free ePanel event featuring pioneers in lipid nanoparticle...
Leveraging Nanoparticle Technologies for Novel Vaccines & Therapies ePanel
Nanoparticles are emerging as a powerful mode of delivery for drugs, vaccines and even gene...